We use a variety of laboratory techniques to figure out absolute ages of rocks, often having to do with the known rates of decay of radioactive elements into detectable daughter products. Unfortunately, those methods don't work on all rocks, and they don't work at all if you don't have rocks in the laboratory to age-date. There's no absolute age-dating method that works from orbit, and although scientists are working on age-dating instruments small enough to fly on a lander I'm looking at you, Barbara Cohen , nothing has launched yet. So that leaves us with relative ages. Relative ages are not numbers.
They are descriptions of how one rock or event is older or younger than another. Relative age dating has given us the names we use for the major and minor geologic time periods we use to split up the history of Earth and all the other planets. Relative-age time periods are what make up the Geologic Time Scale. The Geologic Time Scale is up there with the Periodic Table of Elements as one of those iconic, almost talismanic scientific charts.
Relative Vs. Absolute Dating: The Ultimate Face-off
Long before I understood what any of it meant, I'd daydream in science class, staring at this chart, sounding out the names, wondering what those black-and-white bars meant, wondering what the colors meant, wondering why the divisions were so uneven, knowing it represented some kind of deep, meaningful, systematic organization of scientific knowledge, and hoping I'd have it all figured out one day. This all has to do with describing how long ago something happened. But how do we figure out when something happened? There are several ways we figure out relative ages. The simplest is the law of superposition: We have no idea how much older thing B is, we just know that it's older.
That's why geologic time is usually diagramed in tall columnar diagrams like this. Just like a stack of sedimentary rocks, time is recorded in horizontal layers, with the oldest layer on the bottom, superposed by ever-younger layers, until you get to the most recent stuff on the tippy top. On Earth, we have a very powerful method of relative age dating: Paleontologists have examined layered sequences of fossil-bearing rocks all over the world, and noted where in those sequences certain fossils appear and disappear.
When you find the same fossils in rocks far away, you know that the sediments those rocks must have been laid down at the same time. The more fossils you find at a location, the more you can fine-tune the relative age of this layer versus that layer. Of course, this only works for rocks that contain abundant fossils. Conveniently, the vast majority of rocks exposed on the surface of Earth are less than a few hundred million years old, which corresponds to the time when there was abundant multicellular life here.
Look closely at the Geologic Time Scale chart , and you might notice that the first three columns don't even go back million years. That last, pink Precambrian column, with its sparse list of epochal names, covers the first four billion years of Earth's history, more than three quarters of Earth's existence.
- Difference Between Absolute and Relative Dating.
- Relative vs. Absolute Time in Geology.
- free affair dating sites australia.
Most Earth geologists don't talk about that much. Paleontologists have used major appearances and disappearances of different kinds of fossils on Earth to divide Earth's history -- at least the part of it for which there are lots of fossils -- into lots of eras and periods and epochs. When you talk about something happening in the Precambrian or the Cenozoic or the Silurian or Eocene, you are talking about something that happened when a certain kind of fossil life was present.
Major boundaries in Earth's time scale happen when there were major extinction events that wiped certain kinds of fossils out of the fossil record. This is called the chronostratigraphic time scale -- that is, the division of time the "chrono-" part according to the relative position in the rock record that's "stratigraphy".
The science of paleontology, and its use for relative age dating, was well-established before the science of isotopic age-dating was developed. Nowadays, age-dating of rocks has established pretty precise numbers for the absolute ages of the boundaries between fossil assemblages, but there's still uncertainty in those numbers, even for Earth. In fact, I have sitting in front of me on my desk a two-volume work on The Geologic Time Scale , fully pages devoted to an eight-year effort to fine-tune the correlation between the relative time scale and the absolute time scale.
The Geologic Time Scale is not light reading, but I think that every Earth or space scientist should have a copy in his or her library -- and make that the latest edition.
What is Absolute Dating
In the time since the previous geologic time scale was published in , most of the boundaries between Earth's various geologic ages have shifted by a million years or so, and one of them the Carnian-Norian boundary within the late Triassic epoch has shifted by 12 million years. With this kind of uncertainty, Felix Gradstein, editor of the Geologic Time Scale, suggests that we should stick with relative age terms when describing when things happened in Earth's history emphasis mine:.
For clarity and precision in international communication, the rock record of Earth's history is subdivided into a "chronostratigraphic" scale of standardized global stratigraphic units, such as "Devonian", "Miocene", " Zigzagiceras zigzag ammonite zone", or "polarity Chron C25r". Unlike the continuous ticking clock of the "chronometric" scale measured in years before the year AD , the chronostratigraphic scale is based on relative time units in which global reference points at boundary stratotypes define the limits of the main formalized units, such as "Permian".
The chronostratigraphic scale is an agreed convention, whereas its calibration to linear time is a matter for discovery or estimation. We can all agree to the extent that scientists agree on anything to the fossil-derived scale, but its correspondence to numbers is a "calibration" process, and we must either make new discoveries to improve that calibration, or estimate as best we can based on the data we have already. To show you how this calibration changes with time, here's a graphic developed from the previous version of The Geologic Time Scale , comparing the absolute ages of the beginning and end of the various periods of the Paleozoic era between and I tip my hat to Chuck Magee for the pointer to this graphic.
Fossils give us this global chronostratigraphic time scale on Earth. On other solid-surfaced worlds -- which I'll call "planets" for brevity, even though I'm including moons and asteroids -- we haven't yet found a single fossil. Something else must serve to establish a relative time sequence. That something else is impact craters.
Earth is an unusual planet in that it doesn't have very many impact craters -- they've mostly been obliterated by active geology. Venus, Io, Europa, Titan, and Triton have a similar problem. On almost all the other solid-surfaced planets in the solar system, impact craters are everywhere. The Moon, in particular, is saturated with them.
Relative Dating vs. Absolute Dating: What's the Difference?
We use craters to establish relative age dates in two ways. If an impact event was large enough, its effects were global in reach. For example, the Imbrium impact basin on the Moon spread ejecta all over the place. Any surface that has Imbrium ejecta lying on top of it is older than Imbrium. Any craters or lava flows that happened inside the Imbrium basin or on top of Imbrium ejecta are younger than Imbrium. Imbrium is therefore a stratigraphic marker -- something we can use to divide the chronostratigraphic history of the Moon. The other way we use craters to age-date surfaces is simply to count the craters.
At its simplest, surfaces with more craters have been exposed to space for longer, so are older, than surfaces with fewer craters. Of course the real world is never quite so simple. There are several different ways to destroy smaller craters while preserving larger craters, for example. Despite problems, the method works really, really well.
Most often, the events that we are age-dating on planets are related to impacts or volcanism. Volcanoes can spew out large lava deposits that cover up old cratered surfaces, obliterating the cratering record and resetting the crater-age clock. When lava flows overlap, it's not too hard to use the law of superposition to tell which one is older and which one is younger.
If they don't overlap, we can use crater counting to figure out which one is older and which one is younger. In this way we can determine relative ages for things that are far away from each other on a planet.
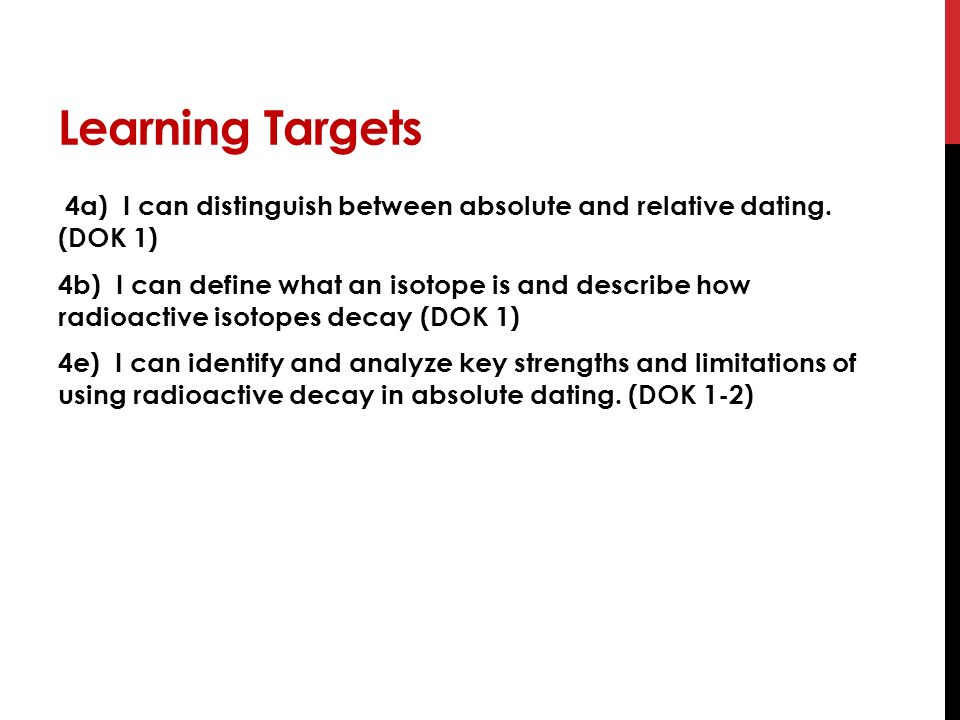
Interleaved impact cratering and volcanic eruption events have been used to establish a relative time scale for the Moon, with names for periods and epochs, just as fossils have been used to establish a relative time scale for Earth. The chapter draws on five decades of work going right back to the origins of planetary geology.
The Moon's history is divided into pre-Nectarian, Nectarian, Imbrian, Eratosthenian, and Copernican periods from oldest to youngest. The oldest couple of chronostratigraphic boundaries are defined according to when two of the Moon's larger impact basins formed: There were many impacts before Nectaris, in the pre-Nectarian period including 30 major impact basins , and there were many more that formed in the Nectarian period, the time between Nectaris and Imbrium. The Orientale impact happened shortly after the Imbrium impact, and that was pretty much it for major basin-forming impacts on the Moon.
I talked about all of these basins in my previous blog post. There was some volcanism happening during the Nectarian and early Imbrian period, but it really got going after Orientale. Vast quantities of lava erupted onto the Moon's nearside, filling many of the older basins with dark flows.
An extended version of stratigraphy where the faunal deposits are used to establish dating. Faunal deposits include remains and fossils of dead animals. This method compares the age of remains or fossils found in a layer with the ones found in other layers.
Relative and absolute ages in the histories of Earth and the Moon: The Geologic Time Scale
The comparison helps establish the relative age of these remains. Bones from fossils absorb fluorine from the groundwater. The amount of fluorine absorbed indicates how long the fossil has been buried in the sediments.
This technique solely depends on the traces of radioactive isotopes found in fossils. The rate of decay of these elements helps determine their age, and in turn the age of the rocks. Physical structure of living beings depends on the protein content in their bodies. The changes in this content help determine the relative age of these fossils.